High Energy and Particle Theory
The goal of high-energy and particle theory research in the Center for Theoretical Physics (CTP) is to enable discoveries of physics beyond the Standard Model (BSM), both through precision tests of the Standard Model itself and through detailed studies of possible new phenomena. With the momentous discovery of the Higgs boson at the Large Hadron Collider (LHC) in 2012, the Standard Model of particle physics is now complete, yet its shortcomings loom larger than ever. For example, the Standard Model cannot account for the nature and origin of dark matter, nor does it address the puzzling hierarchy between the electroweak and Planck scales. On cosmological scales, questions remain about what drives the accelerating expansion of the universe, both today and during the inflationary epoch.
For this reason, high-energy and particle theorists in the CTP are developing new theoretical frameworks to address physics in and beyond the Standard Model. The current effort in the CTP includes research that has a direct impact on experiments as well as research that pursues more formal theoretical directions. CTP researchers study possible new physics signatures at dark matter detection experiments, cosmological observatories, accelerators like the Large Hadron Collider, high intensity experiments, and small-scale table-top devices. At the same time, research in particle theory offers opportunities to push the boundaries of knowledge in quantum field theory (QFT), and innovations and creativity in QFT has long been a theme that unites the research conducted in the CTP.
The CTP has a long history of leadership in high-energy and particle theory. Emeritus faculty Dan Freedman, Jeffrey Goldstone, and Roman Jackiw are responsible for some of the fundamental theoretical ideas – especially those associated with symmetries and symmetry breaking – which lie at the heart of the Standard Model and its extensions. Frank Wilczek is one of the authors of the Standard Model and a pioneer in the study of axions and anyons, with long-standing interests in unification and supersymmetry. Retired faculty Eddie Farhi and Robert Jaffe have taken techniques developed in particle theory and applied them to the fields of quantum computation and fluctuation physics, respectively. Tracy Slatyer and Jesse Thaler represent the next generation of particle theorists, whose work draws on experimental and theoretical developments in areas ranging from dark matter detection to quantum chromodynamics to formal supergravity.
Successful high-energy and particle theorists have an appreciation and understanding of experimental and observational methods. The CTP prides itself on maintaining close connections to experimental research conducted in the Laboratory for Nuclear Science and the MIT Kavli Institute for Astrophysics and Space Research. There are also exciting synergies between the CTP and the NSF Institute for Artificial Intelligence and Fundamental Interactions (IAIFI), where machine learning techniques are being fused with QFT principles to enhance data analysis efforts at the LHC and beyond.
Dark Matter and BSM Model Building
Dark matter is a key research direction in the CTP, bridging particle physics and astroparticle physics (see Cosmology page).
Video by Bill Lattanzi | MIT Center for Theoretical Physics
The gravitational evidence for dark matter is overwhelming, but the nature and origin of dark matter is still unknown. The two leading paradigms for dark matter are axions and massive stable relics (possibly of supersymmetric origin), but given the lack of any conclusive dark matter signals to date, CTP researchers are taking imaginative approaches to dark matter and its potential signatures. Jesse Thaler and Tracy Slatyer have developed innovative models for dark matter in the context of expanded “dark sectors”, where the dark matter interacts with other new particles and forces. These scenarios can produce new and unexpected signals in Earth-based experiments – at particle accelerator laboratories or underground neutrino detectors – as well as in astrophysical and cosmological data. Jesse Thaler proposed the original idea for the ABRACADABRA experiment searching for ultralight axion dark matter, which is led by Lindley Winslow of the Laboratory for Nuclear Science. Frank Wilczek, who invented axions and demonstrated their relevance for dark matter, proposed an plasma haloscope called ALPHA to search for micro-eV axions. Iain Stewart and Tracy Slatyer are collaborating to apply powerful techniques from effective field theory, originally developed to study physics at the Large Hadron Collider, to the case of signals from heavy colliding dark matter particles.
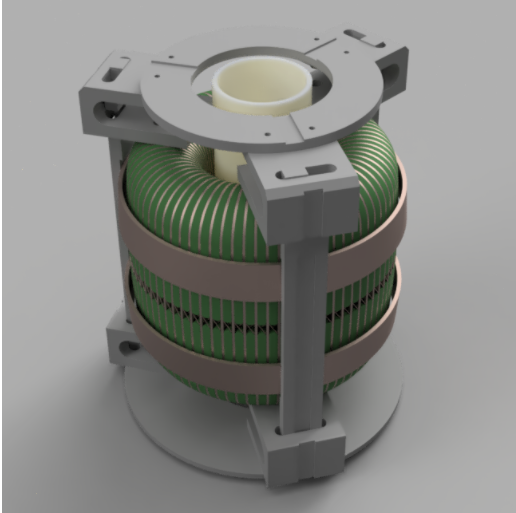
QCD and Collider Physics
Jet physics is an area of continued importance for particle phenomenology, especially at hadron colliders like the LHC. Jets are collimated sprays of particles that arise when quarks and gluons are produced at high energies, and copious jet production is a potential smoking gun for various scenarios beyond the Standard Model. Jesse Thaler has been at the forefront of the emerging field of jet substructure, developing new jet analysis techniques to capitalize on the exceptional ability of the LHC experiments to resolve jet constituents. These jet substructure methods can enhance BSM signals above Standard Model backgrounds, and they are currently being implemented in new physics searches by the MIT CMS pp group. These methods have also revealed fascinating new insights into the dynamics of QCD at high energies. Iain Stewart and Jesse Thaler have developed new techniques to perform precision jet calculations, capitalizing on recent development in applying resummation techniques to hadronic collisions. Jet substructure has offered new probes of the phenomena of jet quenching in the quark/gluon plasma, an area of considerable interest to the MIT CMS heavy ion group. More recently, machine learning and optimal transport techniques have offered new ways to disentangle and visualize jet properties.
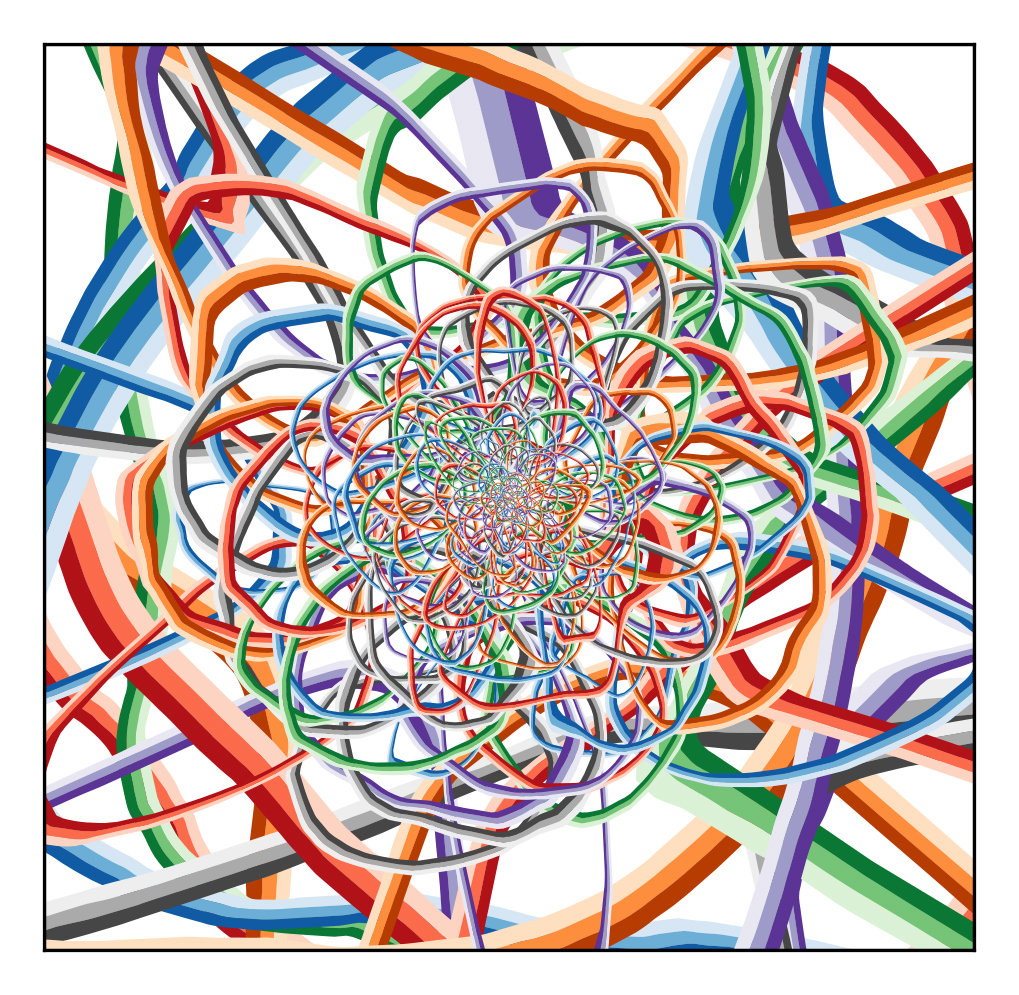
Higgs and Precision Physics
Higgs physics is another area of continued importance, especially with plans for precision Higgs measurements at the high-luminosity LHC and at possible future colliders. Percent-level measurements of the Higgs boson couplings are needed to test the Higgs boson’s role in generating fundamental particle masses. Frank Wilczek has long emphasized that BSM scenarios such as supersymmetry predict small deviations in these couplings as well as additional Higgs particles. Frank Wilczek and Jesse Thaler have shown how the Higgs boson and related Higgs-like states can act as the portal to dark matter. The connection between Higgs physics and BSM physics remains an active area of research. Precision calculations are crucial for studying the detailed characteristics of the Higgs boson, and Iain Stewart has applied effective field theory methods to calculate key Higgs cross sections and thus reduce theory uncertainties in Higgs measurements.
The precision frontier goes well beyond Higgs physics and encompasses the full range of gauge theory dynamics. In the context of QCD, Iain Stewart has used theoretical insights to predict the impact of hadronization on certain jet observables, and Jesse Thaler has shown how multi-point correlators can expose the parton-to-hadron phase transition in LHC data. Other areas of precision investigations in the CTP include electroweak effects, CP violation, and flavor physics.
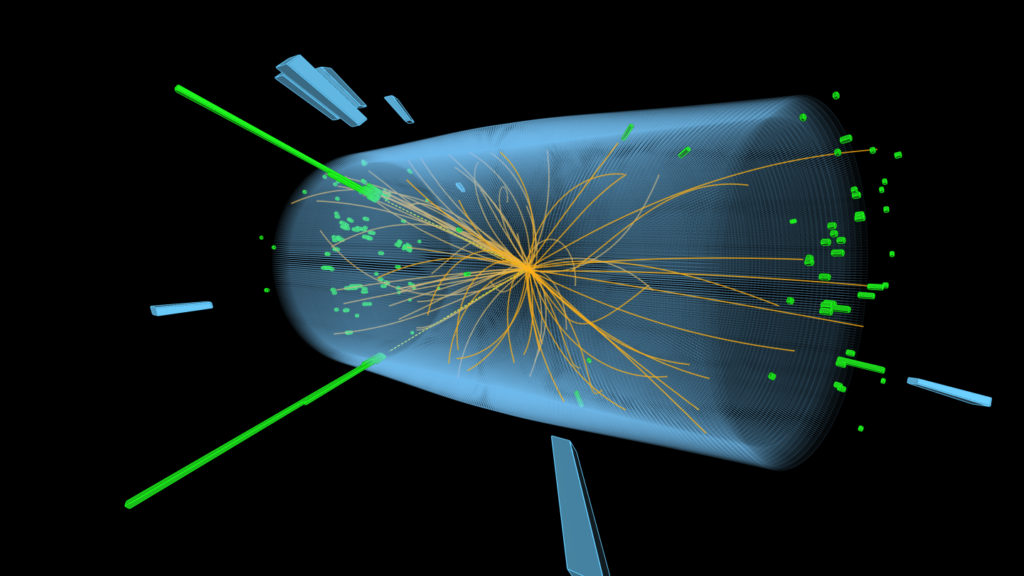
Quantum Field Theory
Particle theory also connects to more formal developments in QFT (as well as string theory). Almost all collider studies involve the calculation of scattering amplitudes, but independent of collider applications, scattering amplitudes themselves have a rich mathematical structure with hidden symmetries. Iain Stewart’s work with effective fields theories has enabled advances in this area, particularly for results beyond the leading order in collinear and soft limits. Supersymmetry is a hypothetical extension of space-time that introduces additional “quantum” dimensions, and many QFT properties are easier to understand in a supersymmetric context. Inspired by potential LHC signatures of supersymmetry, Jesse Thaler has shown that the dynamics of supersymmetry breaking can be richer than previously thought, leading to new results in formal supergravity. Strong dynamics is a feature of many extensions of the Standard Model, and one can gain some analytic handles on these scenarios by treating them as if they were conformal field theories (i.e. special QFTs with a scaling symmetry). Conformal field theories may also be relevant for understanding jet physics, since the interactions of quarks and gluons can sometimes be approximated as having a scaling symmetry. A new understanding of symmetry in quantum field theory is being developed by a combination of high-energy and condensed matter theorists, leading to an improved understanding of phase transitions, anomalies, and strongly-coupled dynamics. Daniel Harlow has made several contributions to this field, including the discovery of a new order parameter for confinement/deconfinement transitions, an improved understanding of the dynamics of the neutral pion in the standard model, and a proof that internal global symmetries which act in nonunitary representations on fields must be spontaneously broken. More generally, techniques developed in particle theory have the potential to offer new insights in other fields.
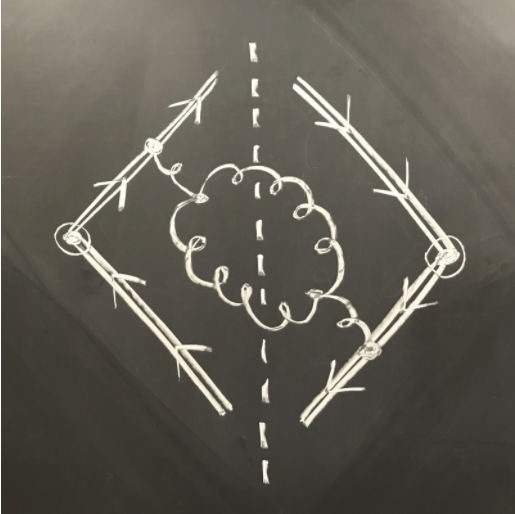
Cosmology and Astroparticle Physics
The interface between particle theory and early-universe cosmology has been a lively area of research since the 1970’s, when physicists realized that hot big bang cosmology would imply that fundamental properties of our universe — from the abundance of chemical elements to maybe also the density of baryons — were determined by high-energy physics processes in the nascent universe. In the present day, our understanding of the universe seems to require “dark energy” and “dark matter” components, which do not have any simple explanation in the Standard Model of particle physics.
Inflationary cosmology, pioneered by Alan Guth in 1980-81, proposed that early-universe particle physics could be responsible for the production of essentially all the matter in the universe, explaining the uniformity of the universe and predicting its average mass density. It was soon discovered that quantum fluctuations during inflation might be responsible for the ripples in the mass density of the early universe—ripples that formed the seeds for structure formation, and which are now visible in the anisotropies of the cosmic microwave background (CMB). The inflationary prediction of the mass density has now been confirmed to an accuracy of about half a percent, and the patterns of ripples seen in the CMB agree very well with the predictions of simple inflationary models.
At the same time, the CMB and other probes have allowed us to measure the amount of dark matter in the universe to percent-level precision. Physicists have established that dark matter must have mass and exert gravity, but it must also be relatively slow-moving, and its interactions (other than gravity) with known particles must be weak or absent. This leaves open a huge range of possibilities for dark matter, from new particles tens of orders of magnitude lighter than even neutrinos, through to primordial black holes formed in the first instants of the universe’s existence.
Mikhail Ivanov uses cosmological large-scale structure to understand dark matter, dark energy, and inflation. The new generation of galaxy surveys will allow for precision tests of these sectors through their imprints on the observed matter distribution. An accurate theoretical understanding of these imprints will be key to harvesting new cosmological information from large-scale structure in this novel regime of high precision.
The cosmology and particle astrophysics program in the CTP focuses on implications for fundamental physics and applications of field-theoretic techniques, complementing the work of our colleagues in the Laboratory for Nuclear Science and the MIT Kavli Institute for Astrophysics and Space Research.
Research on inflation
David Kaiser and Alan Guth are continuing to pursue the connection between particle theory and early-universe cosmology. Much of the work of Kaiser and his group has centered around understanding the dynamics and the predictions of inflationary models that include realistic features from high-energy physics: multiple interacting fields, each with nonminimal gravitational couplings. His group has also developed novel techniques to study the dynamics of inflationary models before and after inflation, including conditions under which the universe may enter an inflationary phase even amid significant inhomogeneities, and the mechanisms by which inflation ends during the “reheating” epoch, when the universe becomes filled with ordinary matter in thermal equilibrium at a high temperature. Alan Guth and collaborators have recently worked on models of inflation at a very low energy scale, and on the cosmology of axions. Guth’s recent research has also included the study of eternal inflation, with the issues it raises concerning the definition of probabilities. Guth and Kaiser are both working with a group of postdocs, graduate students, and undergraduates to study the possibility of the production of primordial black holes in the context of a particular type of inflation, called hybrid inflation.
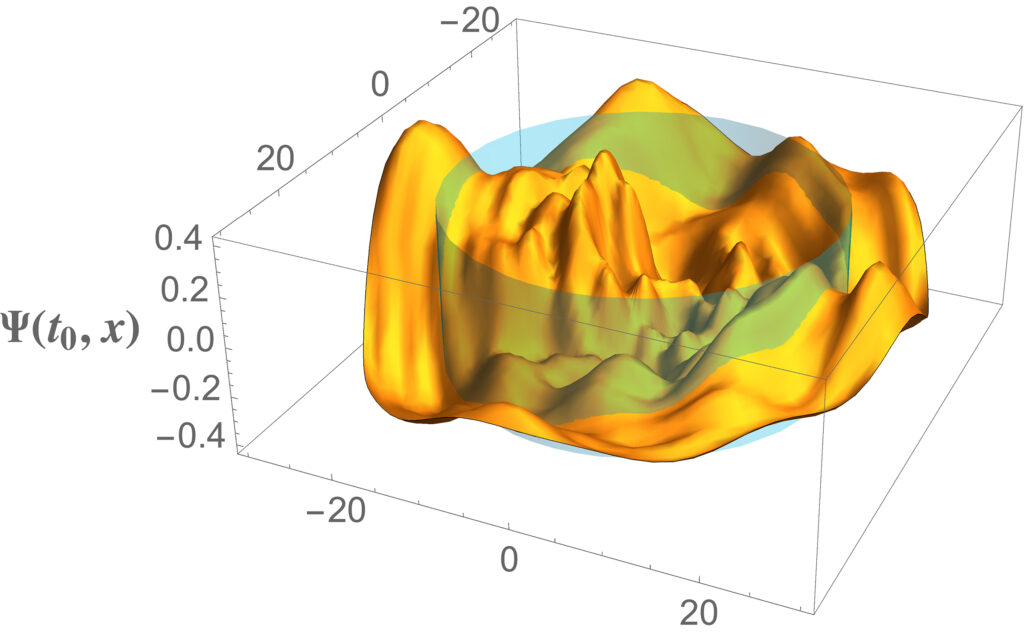
Testing the foundations of quantum mechanics
In a separate line of investigation, David Kaiser and Alan Guth have both been part of the international “Cosmic Bell” collaboration, which has tested the foundations of quantum mechanics by conducting experimental tests of Bell’s inequality using real-time astronomical observations of high-redshift quasars to determine which measurements to perform on entangled particles. Kaiser has also worked with Joseph Formaggio in the Laboratory for Nuclear Science to use data on neutrino oscillations to test the Leggett-Garg inequality across unprecedented length-scales.
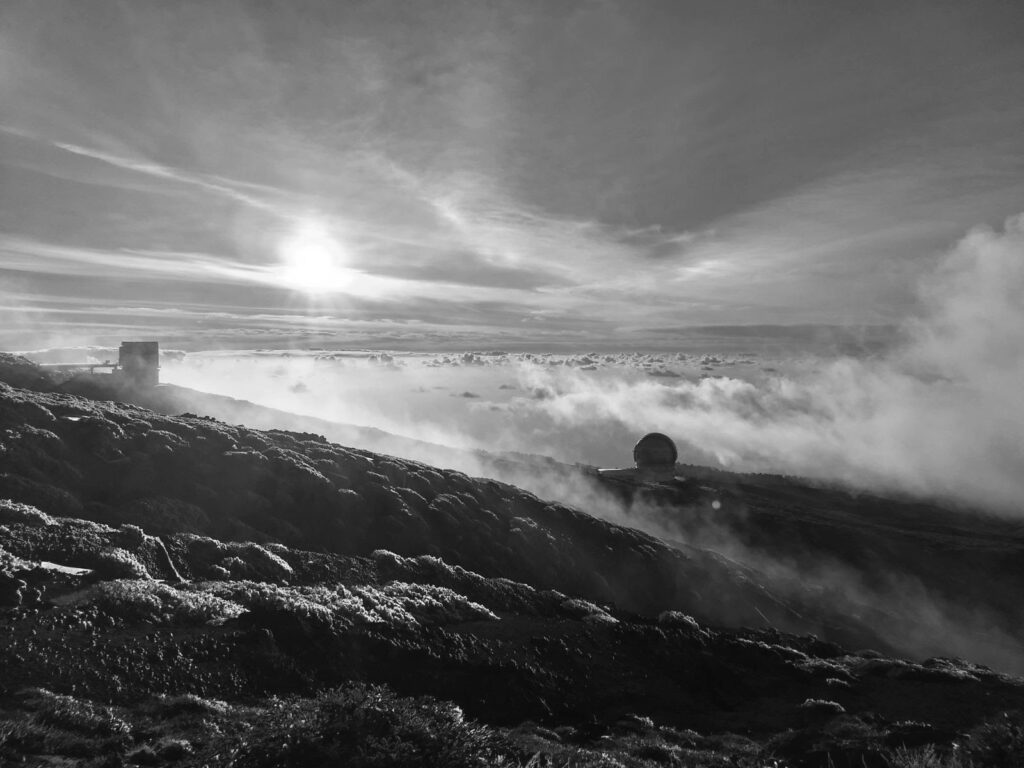
Astrophysical and cosmological dark matter signals
Video by Bill Lattanzi | MIT Center for Theoretical Physics
Annihilations or decays of dark matter could modify the thermal and ionization history of the universe, with possible observational consequences for nucleosynthesis, the cosmic microwave background and the redshifted 21cm line. In the present era, the same phenomena could provide striking signals from regions of high dark matter density. Tracy Slatyer’s group works extensively on the interpretation of such signals, developing new constraints and identifying possible signatures of dark matter physics, from radio to gamma-ray wavelengths, with a particular focus on data from the Fermi Gamma-Ray Space Telescope and signals from the early universe.
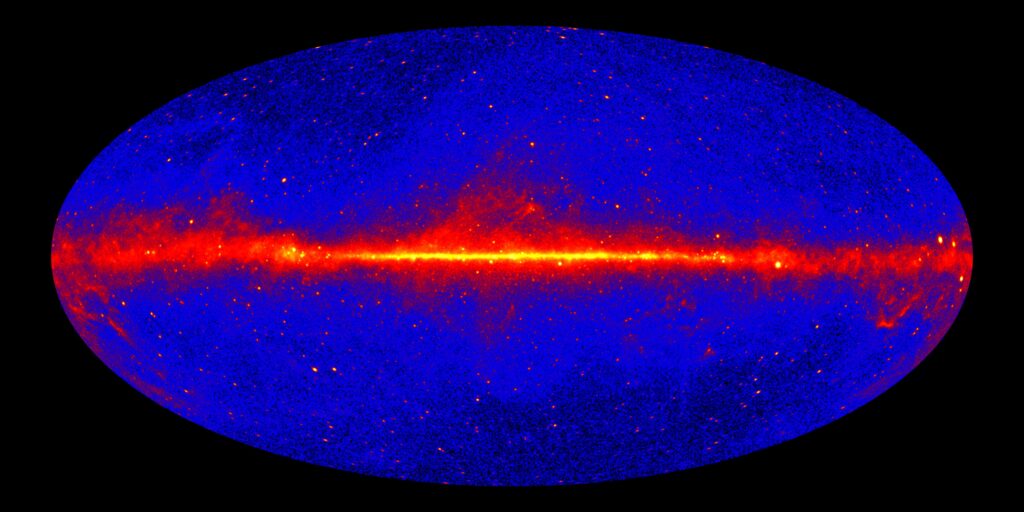