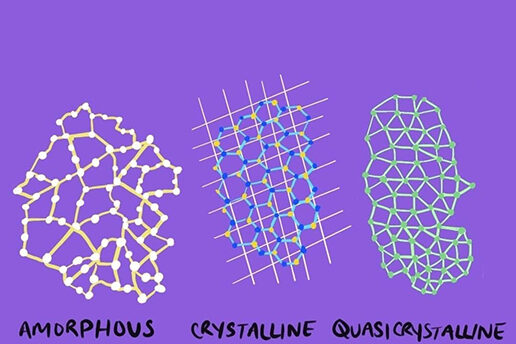
A journey through the history of quasicrystals
U of T and MIT physicists’ recent discoveries about the nature of these structures
Quasicrystals are solids made of conductive metals like aluminum, copper, and iron and are used in many practical items, like non-stick frying pans, needles, and LED lights.
Recently, U of T physics professor and researcher Sergio C. de la Barrera and his colleagues from the Massachusetts Institute of Technology (Aviram Uri, Sergio de la Barrera, Mallika Randeria, Daniel Rodan-Legrain, Trithep Devakul, Philip Crowley, Nisarga Paul, Liang Fu, Raymond Ashoori and Pablo Jarillo-Herrero) discovered a way to create superconductive quasicrystals.
Properties of quasicrystals
Quasicrystals have properties of both crystalline structures, like diamonds, and amorphous structures — structures without an atomically definite form — like glass. Like crystals, the atoms of quasicrystals are organized into patterns. Like amorphous solids, those patterns do not repeat.
Regular crystals exhibit two-fold, three-fold, four-fold, or six-fold symmetry at the atomic level. This means they can be divided into equal halves in two, three, four, or six ways. Quasicrystals can additionally exhibit five-fold, ten-fold, or twelve-fold symmetry.
Because of their unique properties, quasicrystals are both durable like metal and brittle like glass. In metals, the atoms are stacked on top of each other in organized layers, allowing for malleability. If pressure is applied, the atoms can “slip” between each other instead of cracking.
In contrast, glass atoms are spread out unpredictably, making the substance non-malleable. Because the atoms cannot “slip” between each other, too much stress will cause glass to break since it has no other way to release this energy. Quasicrystals have some level of atomic pattern, giving them the durability that metals have, but quasicrystals cannot bend like metals can, making them brittle like glass.
The hunt for quasicrystals
Scientist Dan Shechtman discovered quasicrystals in 1982 while researching aluminum and manganese at the National Institute of Standards and Technology in Maryland, US. Shechtman and his team melted the two metals together and rapidly cooled them back to a solid to create a mixture of metals called an alloy.
After viewing the alloy under an electron microscope, Shechtman noticed it exhibited ten-fold symmetry, writing “10-fold???” in his journal notes. As mentioned prior, this does not happen with crystalline structures like metals.
This is the first known record of quasicrystals. Shechtman later won the Nobel Prize in Chemistry in 2011 for his discovery.
Simultaneously, from the late 1970s to mid-’80s, physicist Paul Steinhardt hypothesized the existence of quasicrystals with five-fold symmetry without knowing about Shechtman’s research. At the time, it was accepted as a fact that crystals could only exhibit two, three, four, or six-fold symmetry. Steinhardt deemed the idea of quasicrystals’ existence “the second kind of impossible,” which became the title of his 2019 book about his journey to prove the existence of naturally occurring quasicrystals.
While hunting to find natural quasicrystals, Steinhardt only encountered two. One was in the Museo de Historia Natural de Florencia in Italy. He and his team traced its origins and found another natural quasicrystal in the Kamchatka Peninsula, Russia. Steinhardt named the quasicrystals icosahedrite and decagonite, respectively.
New discoveries in quasicrystals
Quasicrystals are normally poor electrical conductors, despite being made of conductive metals like aluminum, copper, and iron. Atoms have electron rings around them, and for a material to conduct electricity properly, the outermost rings must have space for electrons to move. Good conductors also need the atoms to be close to each other so that electrons can jump easily from one atom to the next.
Quasicrystals are normally akin to semiconductors, like silicon, tin, and selenium in their conductivity. In a 2023 Nature study, U of T and MIT researchers created ‘superconductive’ graphene quasicrystals, or quasicrystals that allow electricity to pass through with no resistance. Graphene is a material extracted from graphite; it is made of a single layer of rings of carbon.
The researchers applied twistronics to their work to make their semiconducting quasicrystals. Twistronics is a scientific field involving rotating atomically thin layers of materials to manipulate the flow of electrons in the material. This was one of the key elements of the researchers’ discovery.
The U of T and MIT scientists started by twisting three graphene layers and used two twist angles to form two moiré patterns. In photography, moiré is a visual effect where patterns overlap with each other and create a wave appearance. The term moiré in physics refers to an image resulting from two similar or identical patterns overlapping each other.
In this case, it applies to the graphene sheets stacking together. The stacking of graphene sheets gives a moiré visual effect. To the researchers’ surprise, the moiré system they used created a quasicrystal. By twisting the graphene, the physicists were able to alter the electronic system of the material such that its conductivity changed.
The researchers then tuned the ‘moiré quasicrystal’ to make it superconductive, which means it can transmit current with no resistance at specific temperatures.
After thorough research on the phenomena, the group believes they are gaining a stronger grasp on the new superconductive quasicrystals. Their research expands what is known about quasicrystals, and how they can be used to better conduct electricity. Future applications could extend to camouflage technology, and thanks to the new research from U of T and MIT, to improving electronic devices.